Manipulating ferroelectric domains in perovskite crystals boosts bulk photovoltaic effect efficiency by 35%
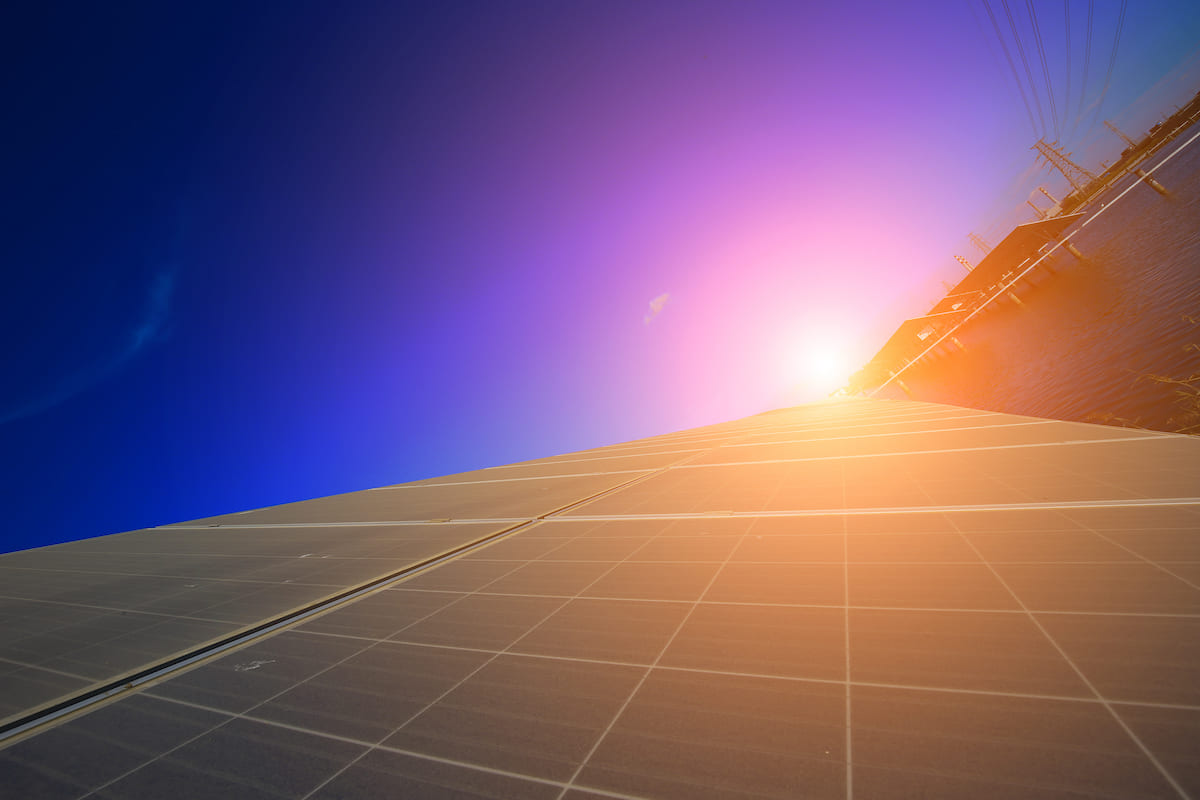
New advancements in solar energy are emerging—not in the traditional semiconductor sector, but in ferroelectric materials that exhibit the so-called bulk photovoltaic effect.
A research team at the University of Oulu in Finland has demonstrated that applying an AC polarization field can significantly enhance electricity generation in these materials. This breakthrough could pave the way for multifunctional solar cells that outperform today’s technology.
What is the bulk photovoltaic effect?
The bulk photovoltaic effect (BPVE) is a physical phenomenon observed in certain non-centrosymmetric materials, such as ferroelectrics, whose crystal structure lacks an inversion center. In other words, these materials do not have a central symmetry point where each atom’s position has an equivalent counterpart on the opposite side.
How does it work? When light is absorbed, it induces an electron flow, generating an electric current without requiring the p-n junction typical of conventional photovoltaics. In BPVE, photoexcited charge carriers inherently possess momentum due to spontaneous polarization—the intrinsic separation of positive and negative charges within the ferroelectric crystal structure.
Bulk photovoltaic effect: advantages and challenges
Studied since the 1960s, this effect theoretically provides a strategy to surpass the maximum efficiency of single-junction p-n solar cells.
“In conventional solar cells, energy harvesting and conversion rely on forming semiconductor p-n junctions,” explains associate professor Yang Bai, who led the research at the Finnish university. BPVE, on the other hand, “creates its own ‘self-junction’ and could theoretically exceed the Shockley-Queisser limit, which restricts the efficiency of single-junction p-n solar cells.”
However, in practice, improving BPVE efficiency to match semiconductor-based photovoltaics remains a challenge. One of the key obstacles lies in material design: achieving high voltage requires thick materials to maximize electrode spacing, whereas achieving high current density necessitates thin materials to minimize energy loss.
This contradiction between the requirements for high voltage and high current keeps the power output of bulk photovoltaic effect-based cells relatively low.
Stacked domain structure: a 35% boost in efficiency
Bai’s team has demonstrated a 35% improvement in the electrical output of these cells by using a stacked domain structure. A domain is a submicrometer-sized region where spontaneous polarizations align in the same direction and can be switched by applying an external electric field.
“This configuration,” the researchers explain, “allows for a large electrode distance when incident light illuminates the film’s surface area.” This helps generate a high open-circuit voltage. At the same time, photocurrent generation remains efficient within each domain wall due to reduced thickness.
Stacked subdomains had previously been theorized for a specific material (a thin film of BiFeO3) but were difficult to verify in other materials due to the complex manipulation of domain walls.
The scientists overcame this hurdle by applying an AC polarization field, which better aligns the microstructure (domains) within ferroelectric crystals compared to the conventionally used DC field. After removing the electric field, the domains remained in a more optimized alignment.
Toward more efficient bulk photovoltaic solar cells
This research paves the way for developing bulk photovoltaic effect solar cells that could unlock new multifunctional applications in photonics, computing, sensing, and energy harvesting.
“The first practical applications will be in small-scale sensing and computing devices, where, in addition to electrical signals, we can introduce light of different wavelengths as an extra degree of freedom for operation,” Bai explains. “For instance, we have already demonstrated BPVE in a filterless color sensor. Other applications include neuromorphic computing components and multi-source energy harvesters for IoT (Internet of Things) devices.“
Despite this breakthrough, further research is needed. The primary challenge now lies in band gap engineering. “Ideally, we need a material with both a narrow band gap (to maximize visible light absorption) and strong spontaneous polarization (to maximize open-circuit voltage).”