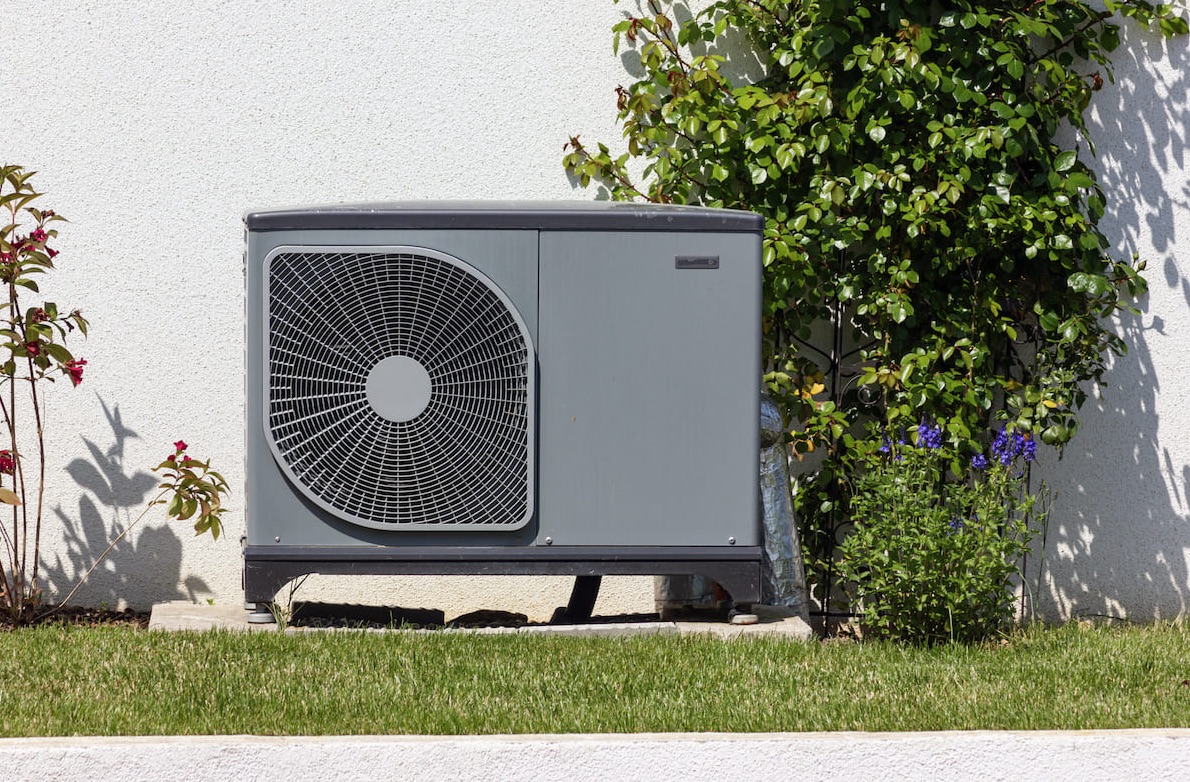
In the world of heat pumps (HPs), various innovative technologies are emerging, aimed at increasing efficiency and reducing costs. One such technology leverages the caloric effect generated by magnetic fields as an alternative to conventional vapor-compression systems. These are magnetocaloric heat pumps, a promising solution for cooling and heating needs.
This emerging technology is the focus of a study conducted by the Ames National Laboratory of the U.S. Department of Energy, which has led to significant advancements. Here, a team of scientists led by Julie Slaughter developed a magnetocaloric heat pump capable of competing with conventional vapor-compression systems in terms of weight, cost, and performance.
The Role of Heat Pumps
With the demand for indoor cooling expected to grow exponentially from now to 2050, reducing the sector’s climate impact has become increasingly urgent. In this context, heat pumps, which leverage electricity and can be powered by renewable energy, represent one of the best technical solutions for reconciling comfort with the fight against emissions.
The traditional version employs a vapor-compression thermodynamic cycle: a refrigerant is compressed or expanded (raising or lowering its temperature) as it circulates between a heat source and a heat sink. While highly effective and widely used, this method is reaching its technical limits in terms of efficiency. Additionally, it has drawbacks, such as potential refrigerant leaks that may result in direct emissions of harmful chemicals.
An alternative under study involves utilizing caloric effects observed under specific conditions in certain solid refrigerant materials. One such effect is the magnetocaloric effect. What is it? It’s a physical phenomenon, particularly evident in certain metals, where temperature changes in response to variations in the magnetic field.
Magnetocaloric Heat Pumps: How Do They Work?
Magnetocaloric heat pumps (MCHPs) harness this effect and are typically based on the concept of the active magnetic regenerator (AMR). In this system, a heat-transfer fluid is actively pumped through a bed of porous magnetocaloric material to transfer thermal energy to heat exchangers. The pump operates by modulating the magnetic field applied to the magnetocaloric material, thus raising or lowering its temperature.
As explained by Julie Slaughter, the magnetic field is typically altered using permanent magnets. “The device’s core involves the rotation of permanent magnets relative to the magnetocaloric material and the use of magnetic steel to contain the field,” the laboratory noted in a press release. “The arrangement of these three components plays a critical role” in determining the system’s performance.
Gadolinium-Based Heat Pumps
The team’s study closely examined two of the most common magnetocaloric materials used in these heat pumps: gadolinium-based materials and lanthanum-iron-silicon hydride alloys.
“In our base device, we kept things simple by using only gadolinium,” said Slaughter. “Lanthanum-iron-silicon materials have a higher power capacity, which naturally increases the achievable density. However, they are not as readily available and require a greater amount of material in the device to achieve good performance.“
The researchers focused on maximizing space efficiency within the heat pump and minimizing the amount of permanent magnetic material and magnetic steel needed for effective operation.
Performance Highlights
“Using finite element models, solid models, and material performance estimates, we predicted thermal power outputs ranging from 37 W to 44 kW at a nominal temperature range of 10 K. Power density is expected to improve from 6 W/kg to 81 W/kg, with an upper limit estimated at 114 W/g,” the team noted.
Compared to the power density of standard compressors under similar ambient temperature conditions, gadolinium-based magnetocaloric heat pumps are competitive up to about 200 W of cooling power. This increases to 1 kW when using LaFeSi alloys and up to 3 kW under optimal conditions.
The research is published in the journal Applied Energy.